Effects of high magnetic field and high hydrostatic pressure on solid-solid phase transformations
Martensitic transformations have been widely exploited to control the microstructure of structural materials. Deep understanding of the transformation is key to develop new structural materials. In our laboratory, we use high magnetic field and high hydrostatic pressure as well as uniaxial stress to understand the kinetics of martensitic transformations. Our purpose is to clarify the mechanism of nucleation and growth mechanism of martensitic transformation.
![]() |
![]() |
Diamond anvil cell. | Martensitic transformation in a Cu-Al-Ni alloy. |
Phase transformation and physical properties of Perovskite-type manganites
Electrical resistivity of some perovskite-type manganites changes drastically in association with its magnetic transition. This characteristic feature has attracted considerable attention by many researchers and has been applied to many devices. In our laboratory, we are investigating the relation between the crystal structure and physical properties such as magnetic and electric properties.
![]() |
![]() |
Magnetoresistance of La0.7Ca0.3MnO3. | Magnetic structure of layered perovskite-type manganites determined by neutron diffraction. |
Large magnetostriction in ferromagnetic shape memory alloys
Some ferromagnetic shape memory alloy exhibits a large magnetostriction of more than 1%. Representative of such alloys are Ni2MnGa, Fe3Pt and Fe-Pd. Our group fond a large magnetostriction in Fe3Pt firstly in the world. Our group also clarified the condition for the appearance of large magnetostriction in ferromagnetic shape memory alloys.
![]() |
Magnetic field-induced strain in ferromagnetic shape memory alloys. |
Large elastic strain realized in alloys exhibiting significant lattice softening
Elastic strain of conventional metals and alloys do not exceed 1%. However, we found that elastic strain of more than 6% can be realized when a single crystal of Fe-Pd and Fe3Pt is compressed in the [001] direction. This behavior is related to the lattice softening of these alloys.
![]() |
Temperature dependence of elastic deformation of Fe-31.2Pd and Fe3Pt in [001] direction. |
Magnetic transition of DyCu and DyAg
Dy is heavy rare earth element with interesting magnetic properties. There is antiferromagnetic coupling between Dy and Cu (Ag), and DyCu and DyAg has triple-q type magnetic structure as shown below. The origin of such a complex magnetic structure arise from quadrupole coupling and other interactions as well as dipole interactions. In our group, we examined magnetization process of DyCu and DyAg, magnetic structure using neutron diffraction, low-temperature specific heat and elastic constants. Using these results, we try to make a quantitative evaluation of quadrupole coupling in DyCu and DyAg.
![]() |
![]() |
Magnetic structure of DyCu in grand state. | Multi-stage metamagnetic transition of DyCu |
Computational materials design based on first-principles calculations
In our Lab., based on first-principles calculations, we investigate (1) phase stability of metals, alloys, compounds and ceramics, (2) spintronics materials and (3) energy-related (e.g., photovoltaic solar cell) materials, and try to reveal the origin of their functionalities. Moreover, we develop our calculations to design new materials based on the obtained knowledge on the electronic structure.
The shape memory effect, which is typically observed in Fe-Pt, Fe-Pd and Ti-Ni alloy systems, originates in the martensitic transformation. By using the first-principles calculations, we can calculate total energy of these alloys as a function of tetragonal distortion (Fig. (1) left panel). By analyzing the calculated electronic structure (DOS, Fermi surface …), we can discuss the electronic origin of the martensitic transformation. As shown in Fig. (1) right panels, we can see the nesting of the Fermi surface in Ti-Ni alloy and it is suggested that this nesting is the electronic origin of the transformation.
Semiconductors are normally non-magnetic material and do not react magnetic field remarkably. However, we can fabricate ‘magnetic semiconductor’ by doping magnetic impurities (e.g., Mn) into the host semiconductors. This magnetic semiconductor is considered as a candidate material for semiconductor spintronics. We have developed first-principles method for estimating Curie temperature of magnetic semiconductors and succeeded to calculate Curie temperature of several systems (Fig. (2) left panel). Moreover, we have developed multi-scale simulation method for reproducing the nano-structure formation in magnetic semicoductors (Fig. (2) right panel). This method might open the possibility of computational materials design by controlling structure formation in materials.
First-principles calculations are now well accepted as reliable methods for predicting material properties of various systems. However, at the same time, its limitation is realized. For example, the local density approximation (LDA), which is one of the standard first-principles methods, underestimates band-gap energy of semiconductors significantly and we cannot apply the LDA for designing photovoltaic solar cell materials. Obviously, we need to have advanced method and we have a collaborative work for the development of electronic structure method. In Fig. (3) left panel, calculation results of band-gap energy of typical semiconductors by using the Quasi-particle Self-consistent GW (QSGW) method developed by Kotani et al., and it is found that the QSGW gives reasonable estimation of the band-gap energy. Based on these calculation, we also estimate conversion efficiency limit by using the Shockley-Queisser theory (Fig. (3) right panel).
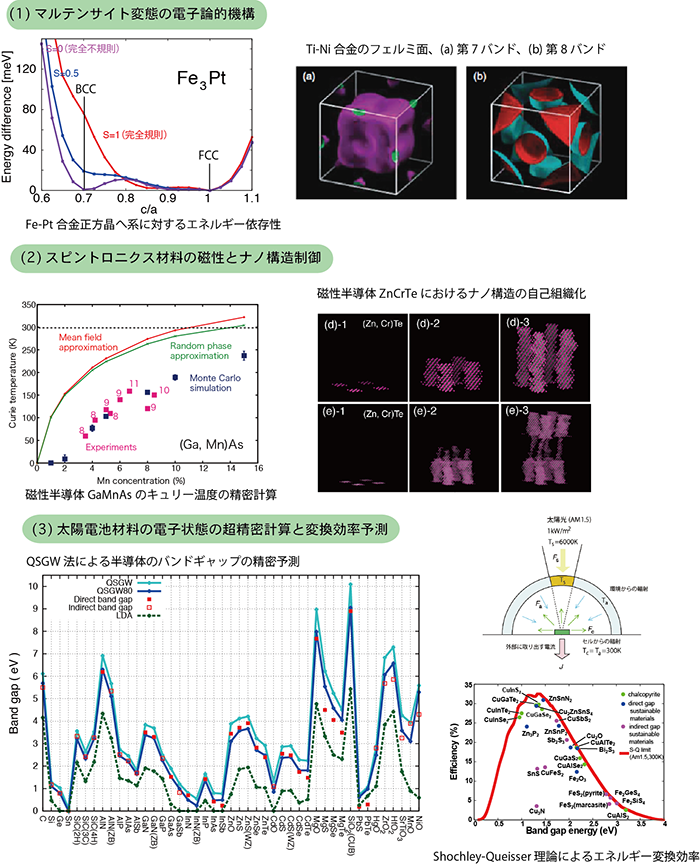
Physical properties at extreme conditions
Structural materials are widely used under various conditions. In near future, it could be used under extreme condition such as very high pressure, high magnetic field etc. We have to understand influence of such extreme conditions on structural materials in order to use them safely. The figure shown below is the change in microstructure of SUS304 stainless steel while holding at 103K. SUS304 stainless steel is widely used for its excellent mechanical and corrosion resistant properties. However, we have to be conscious that the austenite phase of SUS304 is not stable under cryogenic temperatures temperature. The lower panel demonstrate that SUS304 is undergo phase transformation when it is held long time at 103K.
![]() |
Isothermal martensitic transformation in SUS304 steel while holding at 103K. |
Microstructure control using magnetic field
Recent development of superconducting technology enables us of using high magnetic field on materials processing. We have been examining influence of magnetic field on phase diagram. Up to now if is clarified that the austenite-ferrite transformation temperature of iron-based alloys increases by the application of magnetic field as shown in the figure below. Also, it is found that microstructure of CoPt and FePt alloys changes drastically when magnetic field is applied during the heat-treatment.
![]() |
Influence of magnetic field on austenite-ferrite transformation temperature in Fe-Rh alloys. |